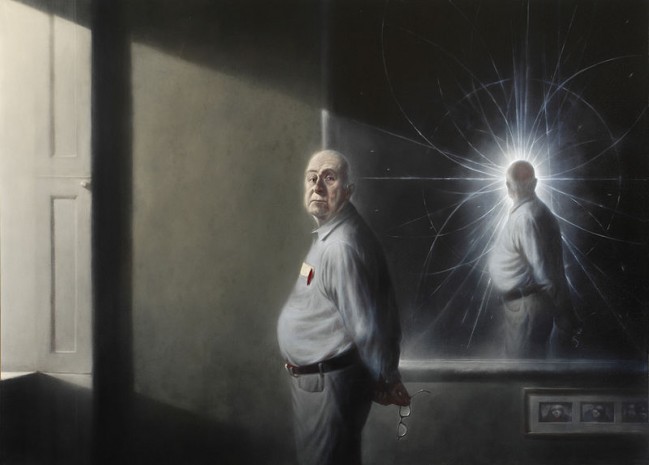
A fascinating profile of Peter Higgs, the theoretical physicist whose name has become associated with the most significant scientific finding of recent times.
From the Guardian:
For scientists of a certain calibre, these early days of October can bring on a bad case of the jitters. The nominations are in. The reports compiled. All that remains is for the Nobel committees to cast their final votes. There are no sure bets on who will win the most prestigious prize in science this year, but there are expectations aplenty. Speak to particle physicists, for example, and one name comes up more than any other. Top of their wishlist of winners – the awards are announced next Tuesday – is the self-deprecating British octagenarian, Peter Higgs.
Higgs, 84, is no household name, but he is closer to being one than any Nobel physics laureate since Richard Feynman, the Manhattan project scientist, who accepted the award reluctantly in 1964. But while Feynman was a showman who adored attention, Higgs is happy when eclipsed by the particle that bears his name, the elusive boson that scientists at Cern’s Large Hadron Collider triumphantly discovered last year.
“He’s modest and actually almost to a fault,” said Alan Walker, a fellow physicist at Edinburgh University, who sat next to Higgs at Cern when scientists revealed they had found the particle.
“You meet many physicists who will tell you how good they are. Peter doesn’t do that.”
Higgs, now professor emeritus at Edinburgh, made his breakthrough the same year Feynman won the Nobel. It was an era when the tools of the trade were pencil and paper. He outlined what came to be known as the Higgs mechanism, an explanation for how elementary particles, which make up all that is around us, gained their masses in the earliest moments after the big bang. Before 1964, the question of why the simplest particles weighed anything at all was met with an embarrassed but honest shrug.
Higgs plays down his role in developing the idea, but there is no dismissing the importance of the theory itself. “He didn’t produce a great deal, but what he did produce is actually quite profound and is one of the keystones of what we now understand as the fundamental building blocks of nature,” Walker said.
Higgs was born in Newcastle in 1929. His father, a BBC sound engineer, brought the family south to Birmingham and then onwards to Bristol. There, Higgs enrolled at what is now Cotham School. He got off to a bad start. One of the first things he did was tumble into a crater left by a second world war bomb in the playground and fracture his left arm. But he was a brilliant student. He won prizes in a haul of subjects – although not, as it happens, in physics.
To the teenage Higgs, physics lacked excitement. The best teachers were off at war, and that no doubt contributed to his attitude. It changed through a chance encounter. While standing around at the back of morning assembly Higgs noticed a name that appeared more than once on the school’s honours board. Higgs wondered who PAM Dirac was and read up on the former pupil. He learned that Paul Dirac was a founding father of quantum theory, and the closest Britain had to an Einstein. Through Dirac, Higgs came to relish the arcane world of theoretical physics.
Higgs found that he was not cut out for experiments, a fact driven home by a series of sometimes dramatic mishaps, but at university he proved himself a formidable theorist. He was the first to sit a six-hour theory exam at Kings College London, and for the want of a better idea, his tutors posed him a question that had recently been solved in a leading physics journal.
“Peter sailed ahead, took it seriously, thought about it, and in that six-hour time scale had managed to solve it, had written it up and presented it,” said Michael Fisher, a friend from Kings.
But getting the right answer was only the start. “In the long run it turned out, when it was actually graded, that Peter had done a better paper than the original they took from the literature.”
Higgs’s great discovery came at Edinburgh University, where he was considered an outsider for plugging away at ideas that many physicists had abandoned. But his doggedness paid off.
At the time an argument was raging in the field over a way that particles might gain their masses. The theory in question was clearly wrong, but Higgs saw why and how to fix it. He published a short note in September 1964 and swiftly wrote a more expansive follow-up paper.
To his dismay the article was rejected, ironically by an editor at Cern. Indignant at the decision, Higgs added two paragraphs to the paper and published it in a rival US journal instead. In the penultimate sentence was the first mention of what became known as the Higgs boson.
At first, there was plenty of resistance to Higgs’s theory. Before giving a talk at Harvard in 1966, a senior physicist, the late Sidney Coleman, told his class some idiot was coming to see them. “And you’re going to tear him to shreds.” Higgs stuck to his guns. Eventually he won them over.
Ken Peach, an Oxford physics professor who worked with Higgs in Edinburgh, said the determination was classic Peter: “There is an inner toughness, some steely resolve, which is not quite immediately apparent,” he said.
It was on display again when Stephen Hawking suggested the Higgs boson would never be found. Higgs hit back, saying that Hawking’s celebrity status meant he got away with pronouncements that others would not.
Higgs was at one time deeply involved in the Campaign for Nuclear Disarmament, but left when the organisation extended its protests to nuclear power. He felt CND had confused controlled and uncontrolled release of nuclear energy. He also joined Greenpeace but quit that organisation, too, when he felt its ideologies had started to trump its science.
“The one thing you get from Peter is that he is his own person,” said Walker.
Higgs was not the only scientist to come up with the theory of particle masses in 1964. François Englert and Robert Brout at the Free University in Brussels beat him into print by two weeks, but failed to mention the crucial new particle that scientists would need to prove the theory right. Three others, Gerry Guralnik, , Dick Hagen and Tom Kibble, had worked out the theory too, and published a month later.
Higgs is not comfortable taking all the credit for the work, and goes to great pains to list all the others whose work he built on. But in the community he is revered. When Higgs walked into the Cern auditorium last year to hear scientists tell the world about the discovery, he was welcomed with a standing ovation. He nodded off during the talks, but was awake at the end, when the crowd erupted as the significance of the achievement became clear. At that moment, he was caught on camera reaching for a handkerchief and dabbing his eyes. “He was tearful,” said Walker. “He was really deeply moved. I think he was absolutely surprised by the atmosphere of the room.”
Read the entire article here.
Image: Ken Currie, Portrait of Peter Higgs, 2008. Courtesy of Wikipedia.